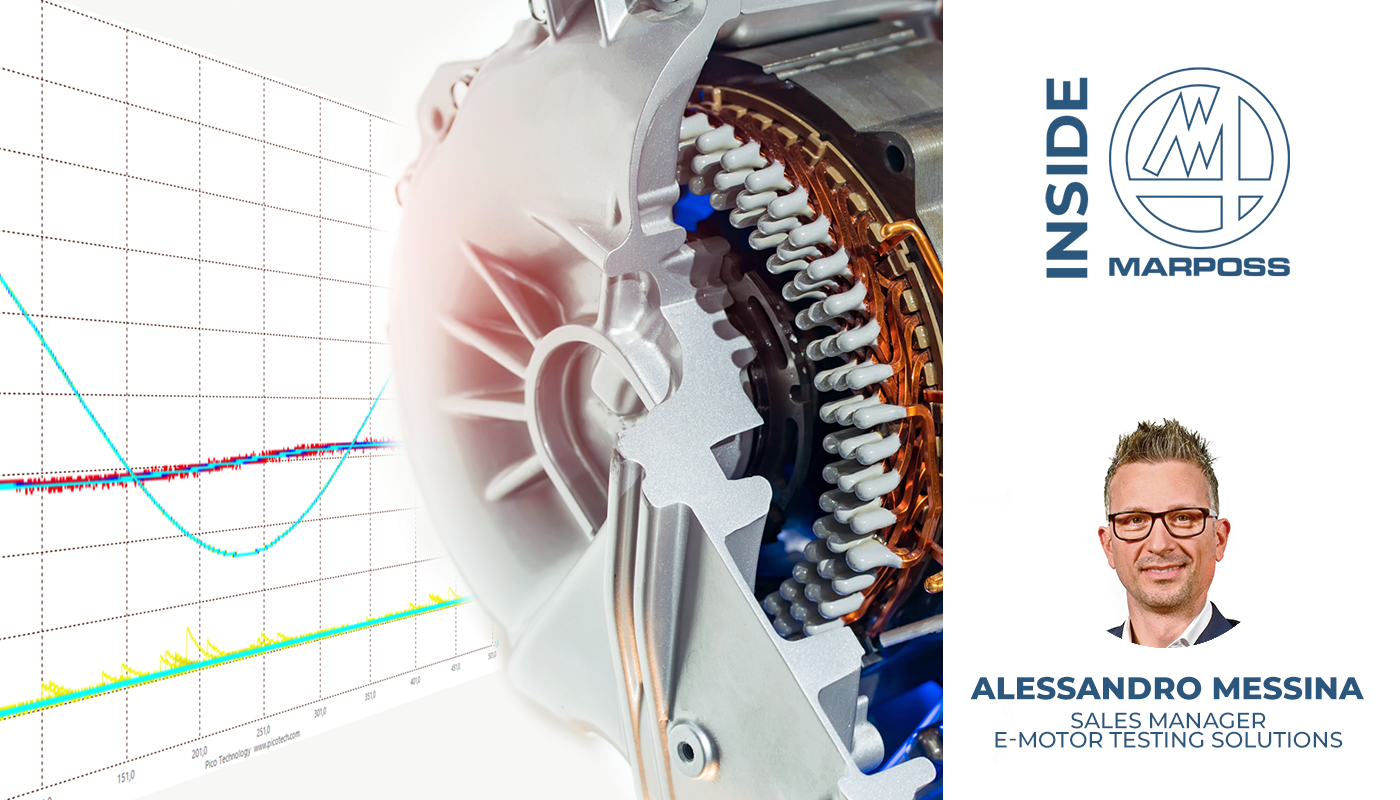
自動車業界では内燃機関から電動パワートレインへの移行が進んでおり、電気モーターの生産における品質と工程管理の基準に大きな変化が生じています。電気モーターは自動車用として広く普及しているため、長期的な信頼性を確保することが最も重要です。
ステーターとローターの巻線の絶縁不良を検出することは、モーターの効率と寿命を確保するために重要です。このテストは、設計段階、微調整段階、製造工程の制御のいずれにおいても常に重要な作業です。絶縁不良により、ステーターの同じ相の巻線間、または異なる相の巻線間、または巻線とケース間で短絡が発生する可能性があります。短絡が発生すると、意図しない低抵抗の電流経路が形成されます。これにより、過電流、過熱、そして最終的にはモーター巻線の損傷につながる可能性があります。絶縁不良により、モーターの効率が低下し、誤動作や故障が発生する可能性があります。
このような絶縁欠陥の存在を検出するために、規制ではサージやHI-POTなど特定のテストを実行することを規定しており、最終製品の品質を保証するために、これらのテストは すべての製品に対して実行する必要があります。
ただし、これらのテストではすべての絶縁欠陥を検出できるわけではなく、絶縁の損失が高エネルギー伝達を伴う絶縁の穿孔を引き起こすような欠陥のみを検出できます。HI-POT テストは、時間の経過とともに絶縁の破壊を引き起こす可能性のある潜在的な欠陥を検出するには不十分です。これらの欠陥は、最初は無害に見えますが、特にインバーターで駆動されるモーターでは、壊滅的な故障を引き起こす可能性があります。したがって、これらの潜在的な欠陥を検出する、高感度で信頼性の高いテスト方法が必要です。
高電流を生成できないことから、従来のテストでは検出できない微細な絶縁欠陥が多数あります。ワイヤーまたはヘアピンのエナメルに閉じ込められた小さな気泡、ステータースロットの絶縁紙の小さな裂け目、ステーター自体の巻線の不完全または不十分な樹脂含浸は、短絡を引き起こすほどではない絶縁品質の低下を引き起こします。
これらの欠陥がテスト中に短絡を引き起こす可能性が低い場合、みなさんはなぜわざわざ検出する必要があるのか疑問に思うかもしれません。残念ながら、これらは最も深刻化する、潜在的な欠陥として定義でき、モーターの使用初期段階では一見無害ですが、短時間で絶縁体を完全に破壊するきっかけとなる可能性があります。
絶縁エナメル内の小さな気泡は、例えば 2つのヘアピンの接触面に現れても、少なくとも最初は大量の電流を流すことはできず、微小放電、いわゆる部分放電のみを発生させます。しかし、電圧ピークごとに継続的に繰り返されるこれらの部分放電は、絶縁体を継続的に弱める可能性があり、必然的に絶縁体の破壊につながります。潜在的な欠陥の大きさとモーターの動作条件の厳しさに応じて、数日または数か月の使用後に破壊が発生する可能性があります。
この現象は、すべてのトラクションeモーター(ただしこれに限定されません)などのインバーターで駆動されるモーターで特に顕著であり、パルス振幅の変調によって過電圧が発生し、これが絶縁体の熱的、電気的ストレスをさらに悪化させる可能性があります。
過酷な動作条件に加えて、自動車業界の伝統的で高い品質管理基準が組み合わさって、潜在的な絶縁欠陥の研究と防止が、トラクションeモーターの製造において不可欠な要件となっています。
部分放電試験は、すべての潜在的な欠陥を検出するための信頼性の高い方法です。部分放電試験を使用した絶縁状態の分析は、プロセスの設計および開発段階で最初に行われます。テストはラボモードで実行され、最初の部分放電が発生するまで徐々に電圧を上昇させながらテストを繰り返し、PDIV(部分放電開始電圧)を特定します。モーターの定格使用条件を考慮しながら、設計パラメーター(絶縁材料の選択など)または製造パラメーター(含浸サイクルの期間など)を変更して、部分放電開始電圧に関して十分な安全マージンを確保することが可能となります。さらに、これらのテストを製造ラインで実行することで、定格電圧で部分放電がないことを確認し、最終製品の品質を保証します。
品質およびプロセス管理では、定格試験電圧で部分放電が発生しないことを確認するために、同じタイプのテストを生産ラインで100%繰り返す必要があります。
これまで述べてきたことから、潜在的な絶縁欠陥によって発生する部分放電を、ラボでも生産ラインでも、高い感度と信頼性で検出できるテストを実施することがいかに重要であるかは明らかです。
実際、この種のテストを高精度で実行するためのさまざまな技術がありますが、それらすべてが生産環境においてすべての技術が同じレベルの信頼性を持つわけではありません。
マーポスは、正弦波およびパルス電圧によるテスト中に3相ステーターによって生成される部分放電 (PD) を測定するための3つの代替方法のパフォーマンスを比較しました。マーポスが検討した3つの方法は、パルス電圧によるテストとしてIEC 61934に記載されている方法です。
マーポスが提案するコンデンサ結合方式は、IEC 60270で要求されている方法で、最大400 Hz の周波数の正弦波電圧によるテスト中に測定された部分放電(PD)のレベルを電荷単位(C)で表現できるため、異なる機器で取得した測定値を直接比較できます。部分放電(PD)のレベルは、正弦波電圧試験ではnC(ナノクーロン)、パルス電圧試験ではmV(ミリボルト)で表されます。これは、当社のすべての装置で採用しています。
アンテナ方式は、部分放電(PD)から放射される電磁波放射の測定に基づいています。結果は常にmVで表されます。
高周波電流トランス (HFCT)方式は、部分放電(PD)の結果としてテスト対象デバイスにテスト電圧を供給するケーブル内で発生する電流ピークを検出します。結果は常にmVで表されます。
3つの方式で測定された部分放電(PD)のレベルを直接比較することは困難です。アンテナ方式と高周波電流トランス (HFCT)方式ではmV単位の測定結果で表されるものの、その振幅は各機器の内部動作に依存するためです。何らかの校正手順を実行しない限り、異なる機器間の比較には適していません。
ただし、部分放電開始電圧(PDIV)を基準にすれば、3つの方式の動作を比較できます。PDIVとは、部分放電(PD)レベルがあらかじめ設定された閾値を超える電圧のことを指します。
(※正弦波信号での試験では実効値(rms)、パルス信号での試験ではピーク値で表されます。)先述のとおり、異なる測定結果の絶対値を比較することは困難ですが、測定されたPD信号が背景ノイズと明確に区別できる電圧を比較対象とすることで、より適切な評価が可能になります。
コンデンサ結合方式を採用した当社の機器の代表として、e.d.c. LT400が使用されました。LT400は、新製品の設計段階のラボ試験用として、また最終モデルの生産開始する前の品質管理部門で使用することを目的に設計されています。
また、生産ラインの最終段階でのテストに使用する最適なテストパラメータを選択することが可能です。LT400は、生産ライン用の当社のマシンと同じソフトウェアを搭載しているため、テストパラメータを生産ラインにスムースに移行できます。さらに、LT400はPD テスト用の高電圧発生器としても機能し、高精度かつ信頼性の高い測定を実現します。
この比較分析では、200MHzと500MHzのUHF帯域で動作し、アンテナまたはHFCTのいずれかからの入力を受け入れることで十分な柔軟性を備える計測器を使用しました。結果として得られるアナログ出力は、PDピークが広がるため、その後の処理が簡素化され、正確な PD 測定に役立ちます。2つの計測器で測定された信号を直接比較するために、PCに接続されたPicoscopeデジタルサンプリングスコープを使用して、テスト対象デバイスに適用される高電圧信号とともに両方の信号を取得しました。
これらのテストにより、部分放電の測定に検討した3つの方式(コンデンサ結合方式、アンテナ方式、HFCT方式)は、正弦波電圧とパルス電圧の両方を使用したオフラインテストで基本的に同等の性能を持つことが示されました。特に、コンデンサ結合方式は、アンテナ方式および HFCT方式のアプローチと実際の部分放電に対して同等であることが示されました。
外部の電磁ノイズに対する耐性に関しては、コンデンサカップリングの方が圧倒的に優れた動作を示しました。ノイズに対する影響は極めて小さく、場合によっては完全に無視できるレベルであり、騒音の多い生産現場での運用において極めて重要な特性となります。一方、アンテナ方式とHFCT方式は、その測定原理に起因し、より高いノイズ感度を示しました。これは、これらのセンサーが基礎としている物理現象を考慮すれば、当然の結果といえます。
コンデンサ結合方式のさらなる利点は、テスト システムの簡単かつ堅牢な校正が可能である点にあります。これにより異なる計測器による測定値の比較が容易になります。正弦波電圧テストとパルス電圧テストの両方で校正器が利用可能で、部分放電の事前定義されたレベルに対応する信号を生成します。正弦波電圧テストの場合は nC単位、パルス電圧テストの場合は mV単位で表されます。
信頼性の高い部分放電(PD) 測定ソリューションの需要が高まり続ける中、コンデンサ結合方式は堅牢で多用途なアプローチとして登場し、正確な測定と外部ノイズに対する耐性の強化を実現します。アンテナ方式やHFCT方式などの代替方法は有益なデータを提供しますが、ノイズの影響を受けやすいため、特定の用途に最も適した技術を選択することが重要です。
潜在的な絶縁欠陥を検出することは、トラクション電気モーターの長期的な信頼性を確保する上で不可欠です。部分放電試験は、これらの欠陥を特定し、潜在的な故障を防ぐための高感度で信頼性の高いソリューションを提供します。この試験方法を研究室と生産ラインにも導入することで、メーカーは厳格な品質基準を維持し、自動車用途向けの高耐久な電気モーターを提供することが可能になります。
e.d.c. LT400のような革新的なソリューションは、部分放電(PD) 測定技術の進化を加速させ、電気システムの信頼性と安全性を向上させます。マーポスが提供する最新の技術と手法をeパワートレインの製造工程全体に活用することで、エンジニアと研究者は e-モビリティの限界に挑戦し続けることができます。